How Does The Fluid Mosaic Model Allow For Membrane Transport?
The fluid mosaic model, proposed by S.J. Singer and G.L. Nicolson in 1972, revolutionized our understanding of the structure and function of biological membranes.
The Fluid mosaic model describes the cell membrane as a dynamic, fluid-structure composed of a lipid bilayer with embedded proteins and carbohydrates, and the fluidity of the membrane allows for various transport mechanisms that are essential for cellular function and survival.
Mechanisms Of Membrane Transport In Fluid Mosaic Model
There are several ways the fluid mosaic model helps for membrane transport. Major of them are:
- Passive transport
- Active transport
- Bulk transport
Passive Transport
Passive transport includes:
Simple Diffusion
- Simple diffusion is the movement of molecules from a region of high concentration to a region of low concentration, driven by the concentration gradient.
- Small, nonpolar molecules such as oxygen and carbon dioxide can easily diffuse across the lipid bilayer.
Facilitated Diffusion: Channels And Carriers
- Facilitated diffusion involves the use of transport proteins to move molecules across the membrane.
- Channel proteins form hydrophilic pores that allow specific ions or water molecules to pass through.
- Carrier proteins bind to specific molecules and undergo conformational changes to transport them across the membrane.
Osmosis: Water Movement Across Membranes
- Osmosis is the movement of water across a semipermeable membrane from a region of high water potential to a region of low water potential.
- The fluid mosaic model allows for the presence of aquaporins, which are water channels that facilitate the rapid movement of water molecules across the membrane.
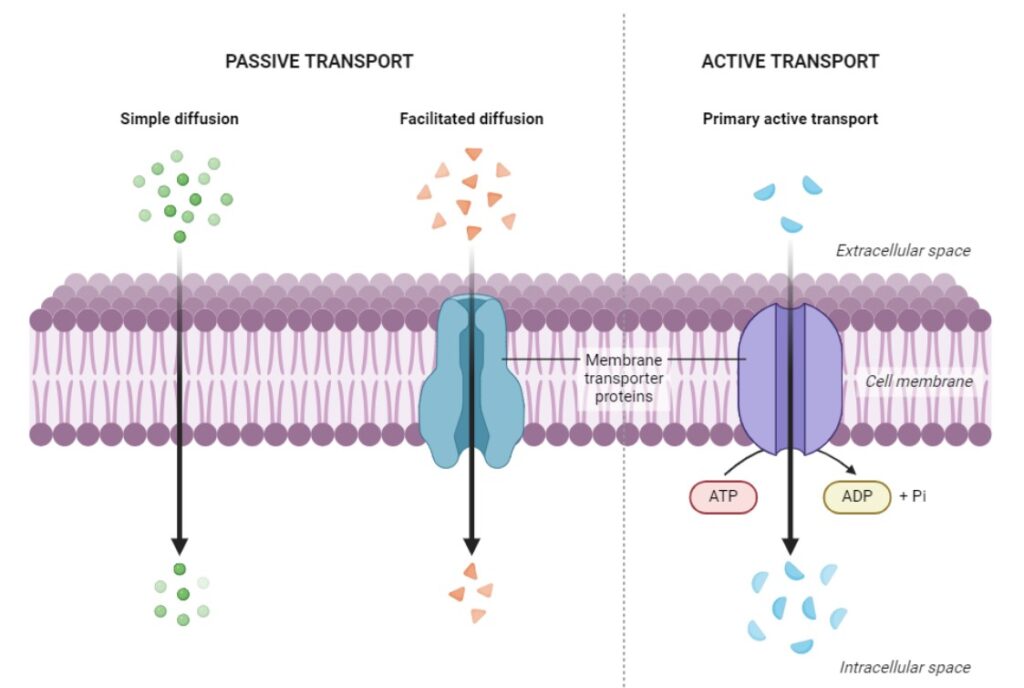
Active Transport
Primary Active Transport: ATP-Driven Pumps
- Primary active transport uses energy from ATP hydrolysis to move molecules against their concentration gradient.
- The sodium-potassium pump is an example of a primary active transport system that maintains the electrochemical gradient across the membrane.
Secondary Active Transport: Symporters And Antiporters
- Secondary active transport uses the energy stored in the electrochemical gradient created by primary active transport to move molecules against their concentration gradient.
- Symporters transport two different molecules in the same direction, while antiporters transport two different molecules in opposite directions.
Electrochemical Gradients And Their Role In Transport
- Electrochemical gradients, created by the unequal distribution of ions across the membrane, provide the driving force for many transport processes.
- The fluid mosaic model allows for the establishment and maintenance of these gradients through the action of ion pumps and channels.
Bulk Transport
Endocytosis: Cellular Ingestion Mechanisms
Endocytosis is the process by which cells engulf extracellular materials by invaginating their plasma membrane.
The fluid mosaic model allows for the formation of endocytic vesicles due to the membrane’s flexibility.
Endocytosis consists of 3 processes:
- Phagocytosis: Engulfing Large Particles Phagocytosis involves the engulfment of large particles, such as bacteria or cell debris, by specialized cells called phagocytes.
- Pinocytosis: Cellular Drinking Pinocytosis is the non-specific uptake of extracellular fluid and dissolved solutes by small vesicles.
- Receptor-Mediated Endocytosis: Specific Uptake Receptor-mediated endocytosis is a selective process in which specific molecules bind to receptors on the cell surface, triggering the formation of clathrin-coated vesicles.
Exocytosis: Secretion Of Cellular Molecules
- Exocytosis is the process by which cells release molecules to the extracellular environment by fusing vesicles with the plasma membrane.
- The fluid mosaic model allows for the fusion of vesicles with the membrane due to its dynamic nature.
Specialized Transport Mechanisms Of Plasma Or Cell Membrane
Aquaporins: Water Channels In Membranes
- Aquaporins are specialized membrane proteins that form water channels
- It allows for the rapid and selective transport of water molecules across the membrane.
- These channels are essential for maintaining water homeostasis in cells and tissues.
Ion Channels
- Ion channels are membrane proteins that form pores selective for specific ions, such as sodium, potassium, and calcium.
- These channels regulate the flow of ions across the membrane, which is crucial for maintaining the electrochemical gradient and generating electrical signals in excitable cells.
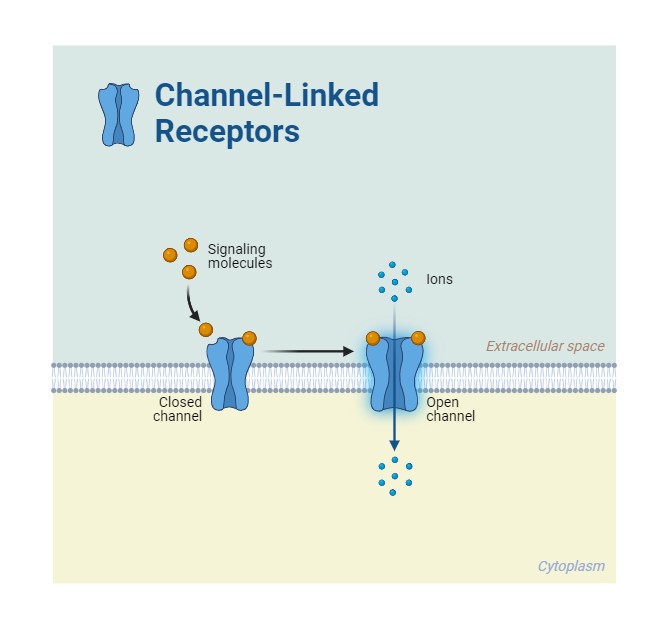
Transporter Proteins
- Transporter proteins are specialized membrane proteins that facilitate the uptake of essential nutrients, such as glucose, amino acids, and vitamins.
- These proteins can operate through various mechanisms, such as facilitated diffusion, primary active transport, and secondary active transport.
Membrane-Associated Processes
Signal Transduction And Membrane Proteins
Membrane proteins play a crucial role in signal transduction, the process by which cells respond to external stimuli.
Receptor proteins embedded in the membrane bind to specific ligands, triggering intracellular signaling cascades that lead to changes in gene expression, metabolism, or cell behavior.
Membrane Fusion and Its Role in Cellular Communication
Membrane fusion is a process by which two separate lipid bilayers merge to form a single continuous membrane. This process is essential for various cellular events, such as exocytosis, endocytosis, and cell-cell communication.
Membrane Lipid Replacement Therapy
Membrane lipid replacement therapy is a novel approach that aims to restore the integrity and function of cellular membranes by supplying exogenous phospholipids.
This therapy has shown potential in treating various conditions associated with membrane dysfunction, such as chronic fatigue syndrome and fibromyalgia.
Structure of the Fluid Mosaic Model
Lipid Bilayer
The lipid bilayer is the foundation of the fluid mosaic model and consists of two phospholipid layers. The hydrophilic heads of this lipid bilayer face the aqueous environment and their hydrophobic tails confront each other.
Cholesterol
Cholesterol is another important component of the lipid bilayer. It helps maintain membrane fluidity and stability by interacting with the phospholipid tails.
Cholesterol also regulates the permeability of the membrane, preventing unwanted substances from entering the cell.
Membrane Proteins
Membrane proteins are embedded within the lipid bilayer and perform various functions:
- Transport proteins: facilitate the movement of molecules across the membrane
- Receptor proteins: receive signals from the external environment
- Enzymatic proteins: catalyze chemical reactions
- Structural proteins: provide support and shape to the membrane
Carbohydrates
Carbohydrates are attached to the outer surface of the membrane, forming glycoproteins and glycolipids. These carbohydrate chains act as identification tags, allowing cells to recognize each other and interact.
Functions of Membrane Fluidity
Impact of Temperature on Membrane Dynamics
Temperature affects the fluidity of the lipid bilayer. At higher temperatures, the phospholipids have more kinetic energy and move more freely, increasing membrane fluidity.
At lower temperatures, the phospholipids are more closely packed, decreasing membrane fluidity.
Influence of Lipid Composition on Fluidity
The composition of the lipid bilayer also influences membrane fluidity. Saturated fatty acids, with their straight hydrocarbon chains, allow for tighter packing and decreased fluidity.
Unsaturated fatty acids, with their kinked hydrocarbon chains, disrupt the packing and increase fluidity.
Cholesterol As A Fluidity Buffer
Cholesterol acts as a buffer for membrane fluidity.
At high temperatures, cholesterol interacts with the phospholipid tails, preventing them from moving too freely and maintaining membrane stability.
At low temperatures, cholesterol disrupts the close packing of phospholipids, preventing the membrane from becoming too rigid.
Advanced Concepts And Recent Developments On Plasma Membrane Fluidity
Membrane Microdomains: Lipid Rafts And Their Functions
Lipid rafts are specialized membrane microdomains enriched in cholesterol and sphingolipids. These domains serve as platforms for signaling molecules and facilitate various cellular processes, such as signal transduction and membrane trafficking.
Membrane Asymmetry And Its Biological Implications
The lipid composition of the inner and outer leaflets of the lipid bilayer is asymmetric. This asymmetry is maintained by the action of flippases and floppases, which actively transport phospholipids between the two leaflets. Membrane asymmetry plays a crucial role in various cellular processes, such as apoptosis and blood coagulation[9].
Cytoskeletal Interactions With The Membrane
The cytoskeleton, a network of protein filaments, interacts with the cell membrane through various membrane-associated proteins. These interactions help maintain cell shape, facilitate cell movement, and regulate membrane transport processes.
Membrane Dynamics And Vesicle Formation
The fluid mosaic model allows for the formation of membrane vesicles, which are essential for various cellular processes, such as intracellular transport and signaling. The dynamic nature of the membrane enables the budding and fusion of vesicles, allowing for the exchange of materials between different cellular compartments.
In conclusion, the fluid mosaic model has revolutionized our understanding of biological membranes and their role in cellular transport.
By providing a dynamic and flexible framework for membrane structure and function, this model has paved the way for numerous discoveries and continues to inspire new research in the field of membrane biology.
Related article: Plant Cell – Definition, Structure, Types, Functions, and Important